Innovation’s role in the evolving nature of economic uranium geology
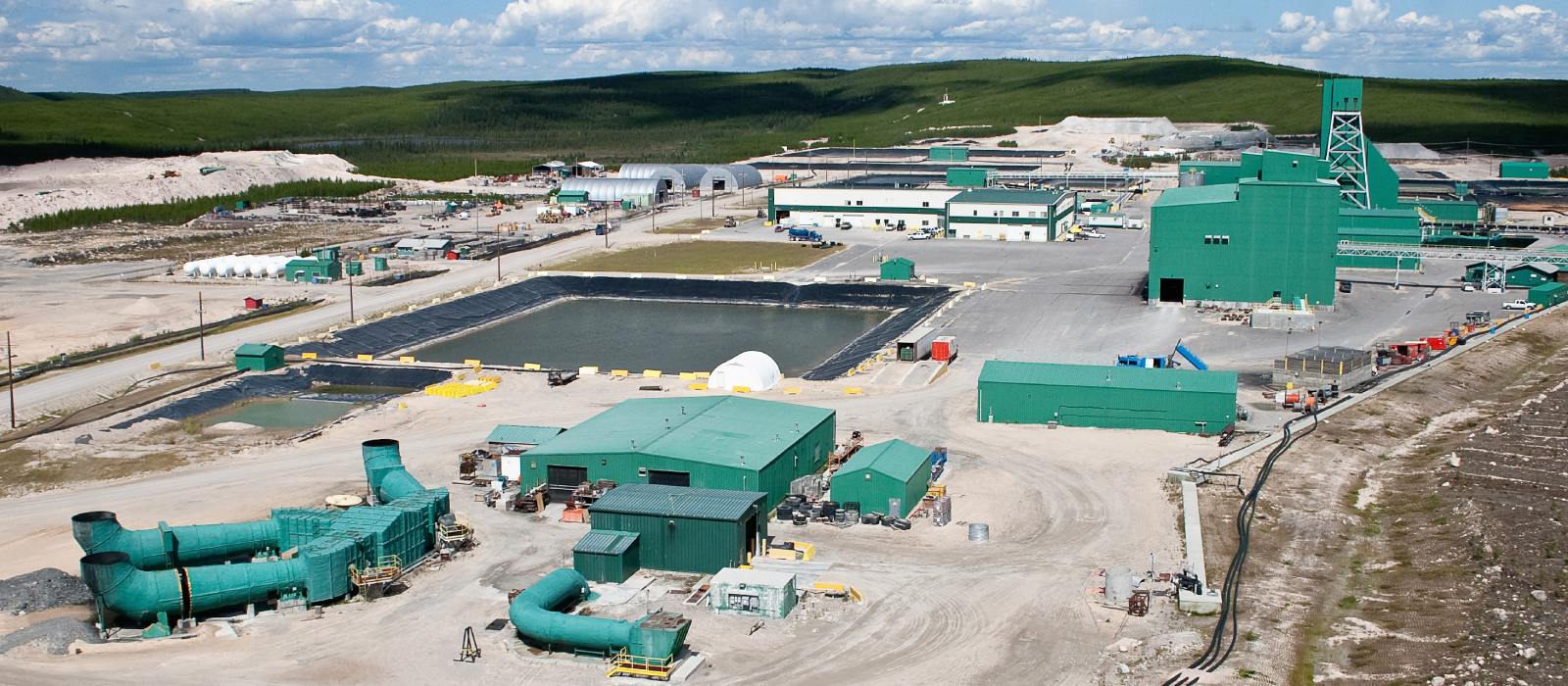
TradeTech was a Premier Content Sponsor of the recent International Uranium Digital Conference 2021.
Uranium is mined on six of the Earth’s seven continents, in multiple jurisdictions, using a variety of extractive technologies.
The geographical distribution of uranium-bearing minerals in the Earth’s crust, combined with a range of conditions that support its economic deposition (Figure 1), distinguish uranium from most other minable commodities. Moreover, the peculiarities associated with economic uranium extraction are precisely what make this industry one of the most challenging sectors to understand and succeed within. And today, the uranium mining industry is shifting.
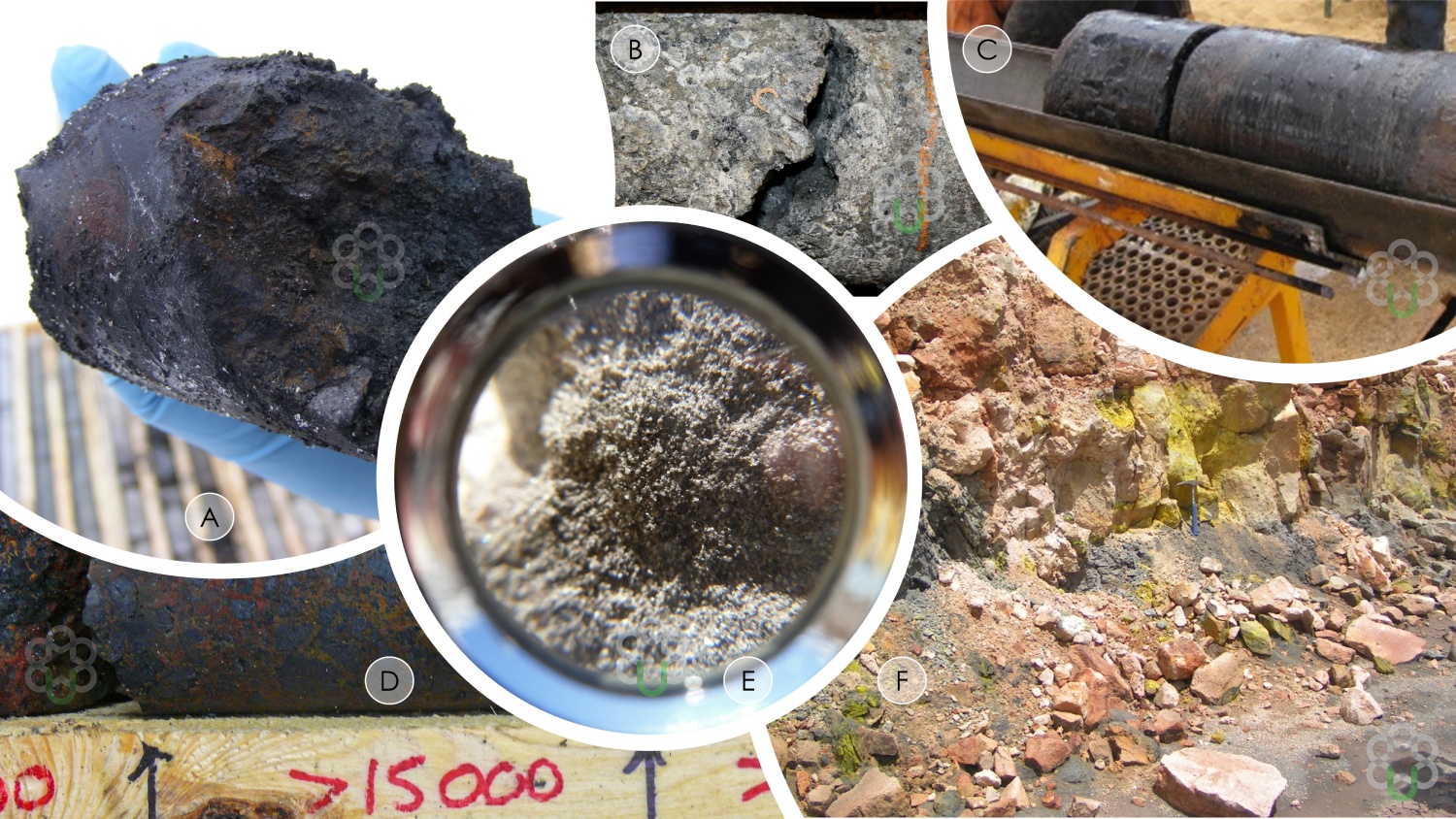
Today, the world’s most widely distributed, and highly regulated, mineral commodity is produced using a range of engineering practices. This includes a process unique to the uranium industry – in-situ recovery (ISR). Combined with oversupply from conventional mines, the inception of large-scale, low-cost ISR operations in Kazakhstan in the early 2000s has had a long-and-lasting impact on uranium prices. Parallel to this, disparate foreign exchange rates across uranium producer regions now manifest as an almost bifurcated national production cost structure; whereby Central Asian producers, such as in Kazakhstan, Uzbekistan, and Russia have an outsized advantage over Australian, Canadian, and US uranium producers.
And therein lies the predicament. Because, while a proportion of new production over the coming decade will undoubtably stem from advanced exploration assets in Kazakhstan and Uzbekistan, the need for end users to maintain a diverse supply portfolio has rarely been more critical than it is today; especially as the world moves towards decarbonised electrification; and especially in a geopolitical landscape becoming increasingly sensitive to trade policies and tensions beyond the control of both nuclear utilities and uranium producers alike.
Unchartered territory or a new normal
Arguably the most successful technology applicable to the uranium extractives industry is that of ISR. First trialled in the US state of Wyoming during the 1960s, ISR has played an increasingly important role in primary uranium production. Through the 1990s, ISR contributed less than 15 per cent of world uranium production. Between 2000 and 2009, contributions from ISR almost doubled to 30 per cent of world output. And, over the last decade, the share of total uranium production attributed to ISR has increased from approximately 34 per cent in 2010 to an estimated 57 per cent in 2020.
Figure 2 shows TradeTech’s monthly U3O8 spot uranium price indicator, the Exchange Value®, alongside the proportion of uranium produced annually using the ISR technique. Bubble size is proportional to the percentage of global production attributable to ISR operations in Kazakhstan. Select events deemed to have an impact on market sentiment and uranium prices are annotated.
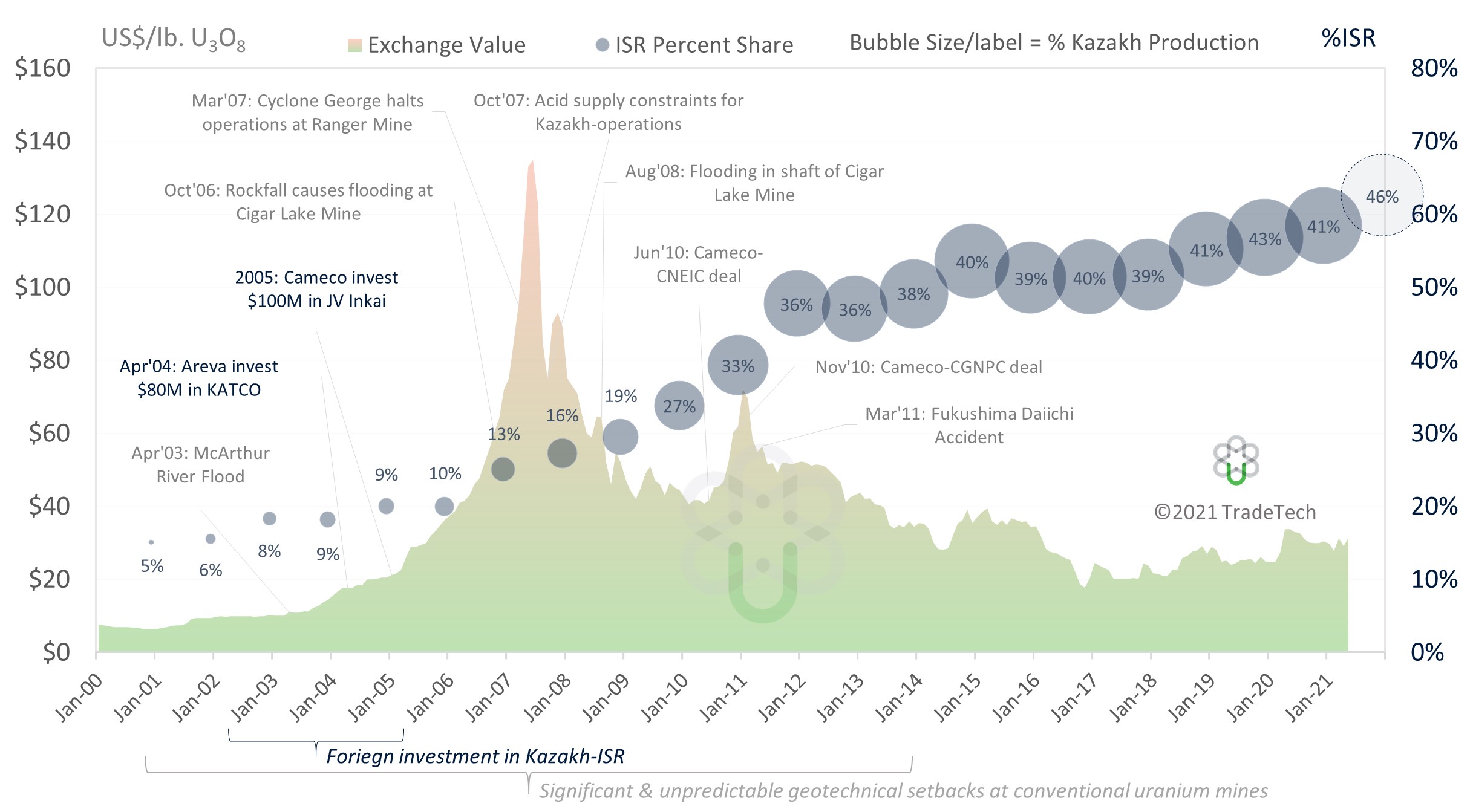
Although uranium prices and ISR output are not directly correlated, since 2009, the increasing proportion of ISR-produced uranium has occurred in concert with steadily decreasing spot prices. Since 2012, ISR has accounted for approximately 54 per cent of global uranium production. Over the last eight-or-so years TradeTech’s Exchange Value has decreased by 38 per cent, from US$52.25 per pound U3O8 in January 2012, to $32.40 per pound U3O8 in June 2021. The decline in uranium prices is a consequence of several factors, one of which is the inception of large-scale and low-cost ISR.
The dramatic increase in ISR output has been led by Kazakhstan’s state-controlled uranium-producing entity National Atomic Company Kazatomprom JSC (Kazatomprom). Kazakhstan’s uranium wealth propelled the nation toward becoming the world’s leading uranium producer in 2013, accounting for approximately 38 per cent of global primary production. In 2015, Kazatomprom launched the company’s Transformation Program. Among its objectives the program assumes the enhancement of production-based efficiencies via the technical re-equipment and replacement of out-of-date equipment coupled with the introduction of modern developments and technologies.
Kazakh uranium production accounted for 41.0 percent of world production in 2018, and 42.3 percent in 2019. Despite the impact of COVID-19 and Kazatomprom’s recent plans to curtail planned production, Kazakh supply is likely to account for most of the global production for the next several years. This success in Kazakhstan is not simply attributable to the nation’s geological endowment.
A trifurcated national production cost structure
The relationship between various domestic currencies and the US dollar can have a profound impact on the financial results of uranium producers, especially since sales of uranium oxide are routinely denominated in US dollars, while production costs are largely incurred in domestic currencies. The relative competitiveness of producer currencies can be examined by translating a hypothetical cost base – say $40 for example – into local currencies in the year 2010, and then reviewing how the US dollar equivalent cost performs over time, as escalated from January 2010.
TradeTech’s Yellow Cake Index (YCI™) incorporates the countering effect of national inflation on exchange rates, which combined generate a clearer reflection of macroeconomic parameters on domestic production costs (Figure 3). Put simply, the YCI™ blends monthly inflation rates with the overall performance of domestic currencies relative to the strength of the US dollar – that is the Australian, Canadian, and Namibian dollar, the Kazakh tenge, Russian ruble, Uzbekistani so’m. and Central African franc (pegged to the Euro). The monthly spot uranium price is also shown for reference.
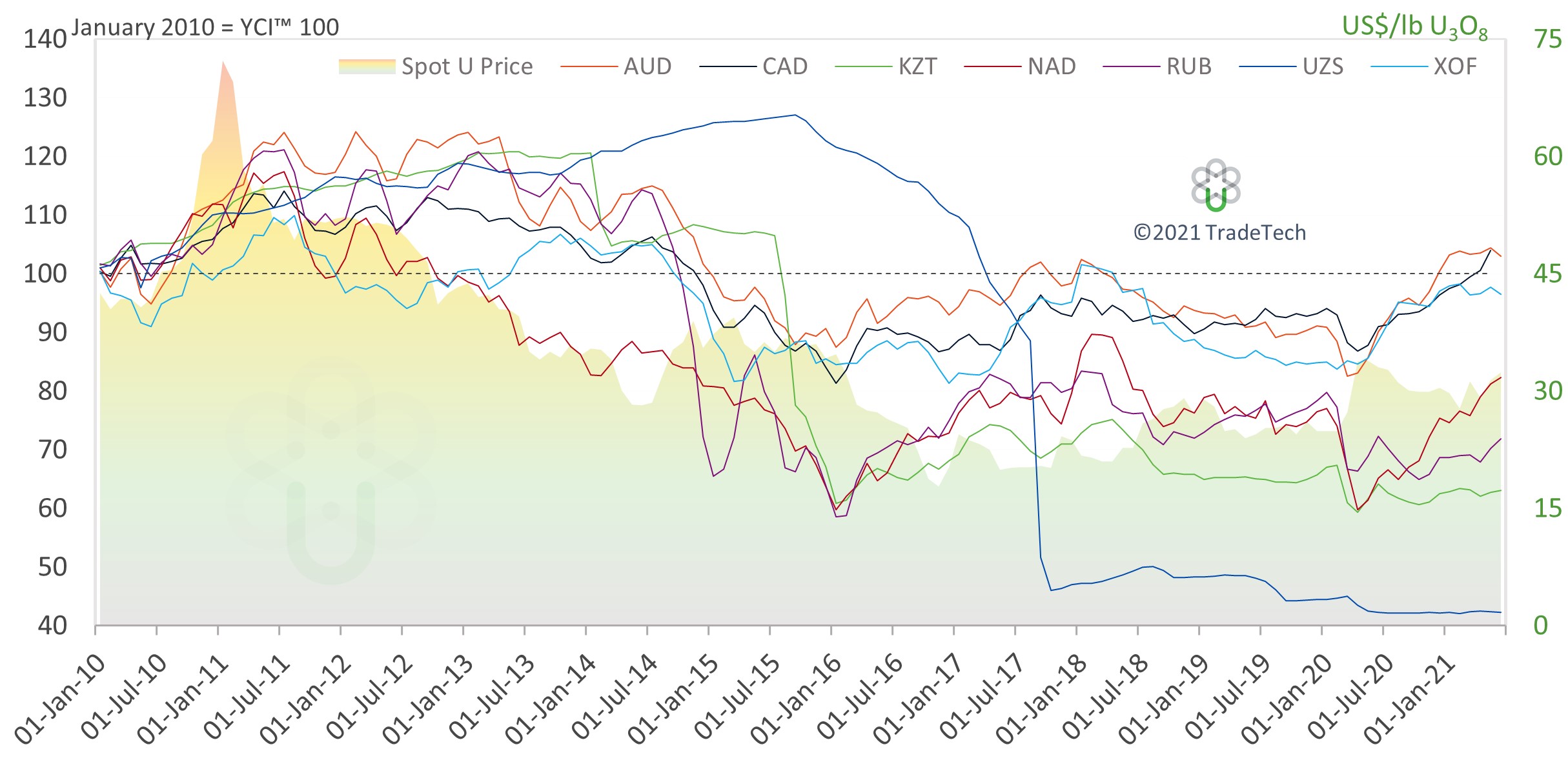
An index value above 100 means that exchange rates and inflation have increased the cost of uranium production in the domestic currency relative to the 2010 US dollar cost equivalent. Conversely, an index below 100 means exchange rates and inflation have improved the cost-competitiveness of domestic uranium production; i.e., production costs have been lowered due to the depreciation of the currency relative to the strength of the US dollar.
The trajectories have separated the producing nations into three distinct camps; the Uzbekistani so’m has received the greatest foreign exchange benefit over the period (since its untethering from the US dollar in September 2017); the Namibian dollar, Russian ruble and Kazakh tenge have received a modest foreign exchange benefit over the period; whereas the Australian and Canadian dollar, and Central African franc have been subject to the greatest domestic cost pressures and received the least foreign exchange benefit.
Figure 3 illustrates just one example of considerations that must be made in determining the economic viability and competitiveness of a uranium resource. Indeed, economic parameters, like foreign exchange rates and national inflation comprise one of the Australasian’s Joint Ore Reserve Committee’s (JORC, 2012) Modifying Factors; that is those considerations used to convert Mineral Resources to economically minable Ore Reserves. These include, but are not restricted to, mining, processing, metallurgical, infrastructure, economic, marketing, legal, environmental, social, and governmental factors.
Innovating beyond the price impasse
(Re)emerging uranium projects have used the time afforded by the protracted lows in the uranium price to invest in innovative technologies with a view toward optimising economic efficiencies and improving their position among an increasingly complex and competitive producer space.
The uranium industry is not new to technological innovation. Cameco Corp’s Cigar Lake operation is the only mine in the world to use the jet boring system (JBS), designed specifically for the technically challenging and high-grade Cigar Lake deposit. The JBS involves using water under high pressure to carve out cavities in the orebody and then collecting the resulting ore slurry through pipes.
The technology took years to implement at Cigar Lake with successive floods occurring in 2006 and 2008 (Figure 2). The Cigar Lake Mine was eventually commissioned in 2014, achieving first commercial production eight years behind schedule and approximately C$1.9 billion (US$1.4 billion) over budget.
A selection of technological innovations being trialled today are outlined in Figure 4. These include applications for open- pit processing technology, underground extraction, first-of-a-kind applications of ISR extraction in new uraniferous lithologies, and by-product production methods.
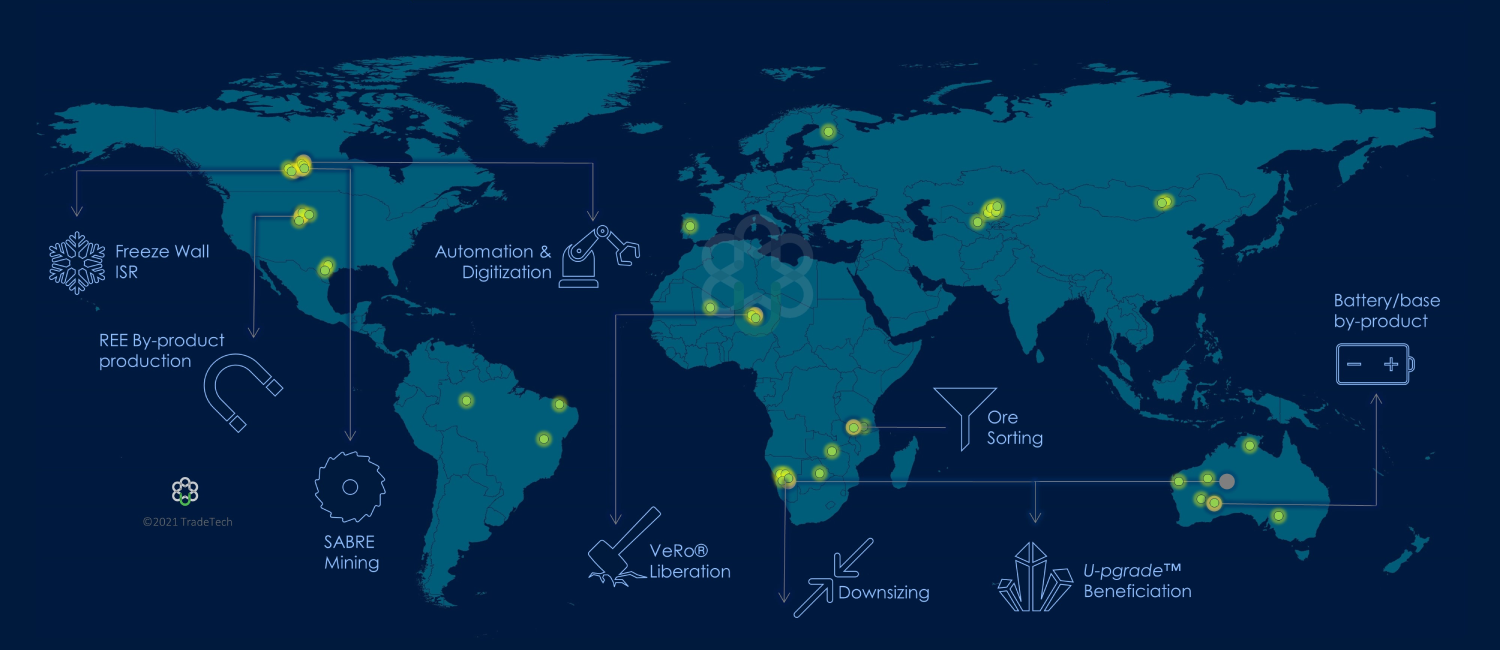
ISR technology meets high-grade Athabascan sandstone: freeze wall technology
Traditional applications of ISR technology have been confined to porous sandstone-type basal and roll-front uranium deposits, mostly in Kazakhstan, South Australia, and the USA. Denison Mines Corp’s asset portfolio is focused in Saskatchewan’s Athabasca Basin, a region well known for its high-grade uranium deposits, occurring at depth, and located at above, or within the unconformity that separates the overlying porous sandstone from underlying crystalline bedrock beneath.
In 2018, Denison revealed plans to exploit the high-grade Phoenix Deposit at its 90 per cent-owned flagship Wheeler River property using ISR technology. Commenting on the company’s Prefeasibility Study in September 2018, President and CEO of Denison David Cates said:
'The selection of ISR mining for the high-grade Phoenix deposit is a defining moment for our Company and a potentially transformational development for the future of uranium mining in the Athabasca Basin—bringing the world’s lowest-cost uranium mining method to the jurisdiction hosting the world’s highest-grade uranium deposits.'
In November 2020, Denison also revealed plans to mine the Tthe Heldeth Túé East pod deposit at the company’s 67-per cent owned Waterbury Lake property using ISR extraction.
The ISR technique uses the native groundwater in the deposit, which is fortified with a low pH solution and in most cases an oxidant. Containment of the mining solution, known as a lixiviant, is a prerequisite to successful ISR operations since this optimises uranium recoveries and minimises regional groundwater infiltration from outside the deposit into the mineralised zone, potentially causing dilution to the lixiviant. In typical ISR operations, this is naturally facilitated through clay or other impermeable geological layers, which makes the geographical distribution of ISR highly regionalised and endemic to specific geological conditions.
At Tthe Heldeth Túé, the crystalline basement rock beneath the mineralised zone provides a natural impermeable layer to contain the lixiviant. However, the sandstone formation that hosts and surrounds the deposit is permeable and is hydraulically connected to the regional groundwater system throughout the Basin. Therefore, to isolate and encapsulate the deposit, Denison has proposed an artificial and impermeable freeze wall that will contain the deposit.
According to Denison, the freeze wall will be established by drilling a series of cased holes from surface and along the perimeter of the deposit, and keyed into the basement rock. Freeze holes will be angled out to surround the mining zone. Circulation of a low-temperature brine solution in the holes will remove heat from the ground, freezing the natural groundwater, and establishing an impermeable frozen wall around the deposit to facilitate the ISR process.
In December 2020, following completion of a trade-off study, Denison also announced a decision to adopt the freeze wall technology for ISR mining at the Phoenix deposit within the Wheeler River Project. In a December 1 announcement, Denison cited advantages that included enhanced environmental design, lower technical complexity, and operational risks, and an expected reduction in initial capital costs with a phased mining approach and strengthened project sustainability.
Underground: Surface Access Borehole Resources Extraction (SABRE) mining method and muon technology
Orano Mining is developing the SABRE mining method, which uses a high-pressure water jet placed at the bottom of the drill hole to recover ore at the surface. Since 2013, Orano has conducted a series of tests and is evaluating its potential for future mining operations. Today, SABRE technology is endemic to Saskatchewan’s Athabasca Basin and is best suited to smaller, high-grade deposits residing around 300 meters from surface and bound by competent host geology.
According to Orano, SABRE can access deposits from the surface using a small footprint, thereby limiting potential environmental impact, and easing the permitting process. A working theory for the deployment of SABRE technology in the Athabasca Basin is via a hub-and-spoke system, like that deployed for ISR operations that use a central facility to process multiple feeds from surrounding satellite wellfields.
Following extraction using the SABRE technology, traditional milling infrastructure is needed to process the ore. Consequently, SABRE’s principal application is realistically confined to the eastern portion of the Athabasca Basin where Orano’s McClean Lake Mill and Cameco’s Key Lake Mill are located. While production from both Cigar Lake and McArthur River Mines would constitute most ore being fed to each respective mill, smaller satellite deposits amenable to SABRE technology and within proximity to each mill could fulfil underutilised (surplus) capacity at each mill. This would enable those facilities to operate toward maximum capacity for longer, driving down milling costs for the owners (Cameco, Orano, and Denison Mines), and reducing toll milling charges for SABRE deposit operators.
Since 2018, the SABRE team has continued engineering and procurement activities to develop bespoke mining equipment and high-pressure pumping systems. This included four access holes at the Orano Canada-operated McClean North deposit. The drilled and cased holes will allow for mining of the orebody during the latter stages of the test mining program, which was originally scheduled to occur in 2020.
Experiential proponents of SABRE technology include, among others, IsoEnergy Ltd and Denison Mines Corp. In 2020, Canadian-based uranium developer Denison, which owns a 22.5 per cent share in the McClean Lake JV, budgeted $4.1 million (US$3.2 million) toward advancing SABRE technology at the McClean Lake and Midwest JV. Most of these expenditures relate to McClean Lake—including $3.6 million (US$2.8 million) in respect of Denison’s share of SABRE related activities.
Parallel to this, in July 2021, Orano announced a partnership with Ideon Technology to deploy the world’s first muon tomography detector for imaging compact uranium deposits in the Basin. Ideon, the proprietors of muon technology, suggest it can provide X-ray-like imaging up to 1 km beneath the Earth’s surface. Multiple borehole muon detectors are deployed down a single drill hole in a connected sequence, delivering progressive imaging results, which feedback to integrated imaging systems, inversion technologies, and artificial intelligence to produce high-resolution 3D density maps of underground targets. Within the context of muon technology, Orano is targeting the imaging of high-grade, compact uranium deposits located at 300 metres depth, which meet the target criteria for SABRE mining.
Near-surface: ore sorting technology
Conventional mining practices, especially those that deploy an open-pit extraction methodology, are materials movement exercises. Following the drill-blast-load-haul sequence, material delivered to the plant will comprise both ore minerals and deleterious waste compounds, known as gangue, which must be sorted and separated ahead of further processing and refining. This process is often referred to as beneficiation.
Australian-based Lotus Resources Ltd, which owns an 85-per cent interest in the idled Kayelekera Mine in Malawi, believes ore sorting technology can positively impact project economics (Figure 5). Ongoing test work using Steinert ore sorting equipment in Perth suggests Kayelekera’s ore, characterized as coarse-grained arkosic units with intervening silty mudstones, is amenable to ore sorting and that high-grade material can be separated from low-grade and/or waste materials. This means the uranium feed grades to the Kayelekera plant could be increased by first passing the ore through an ore sorting unit, removing the lower-grade/waste material and feeding the ‘concentrate’ to the plant.
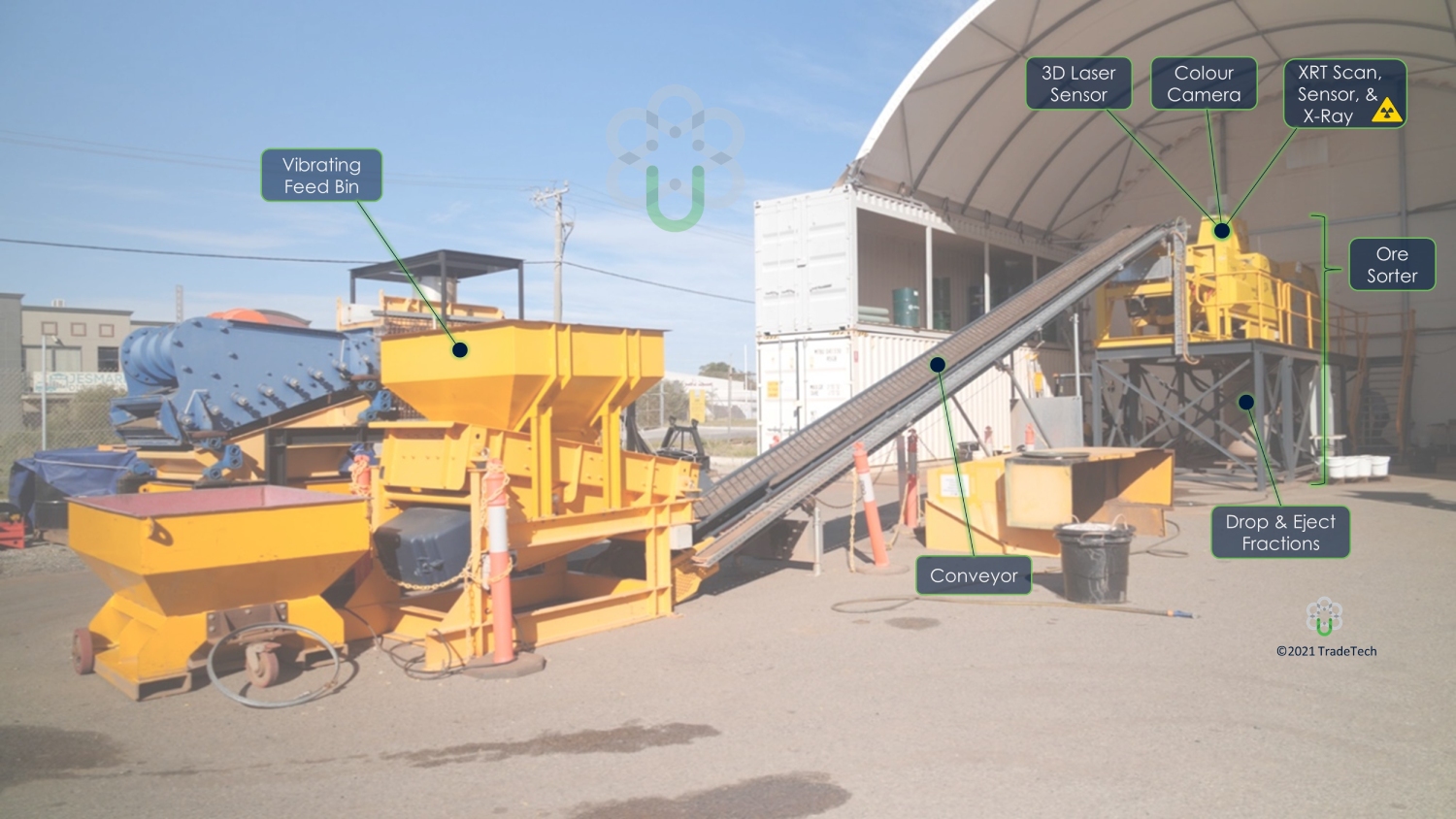
Source: Lotus Resources Ltd.
Between 2007 and 2014, during operations under previous owner Paladin Energy Ltd., marginal low-grade ores were stockpiled and were not included in the production schedule. Lotus is anticipating that the ore sorting technology will be applicable to these ores and will ‘convert’ these marginal ores into more economic ores by rejecting the lower grade component producing a product with similar specifications to the higher-grade direct feed ore. This will increase the life-of-mine (LoM) at relatively low cost as these resources have already been mined and the capital and operating cost of an ore sorting unit is relatively low.
There are two other areas that ore sorting may also benefit the Kayelekera Project; the first is related to acid consumption in the plant. The Kayelekera ores have relatively high acid consumption (50-100kg/t); there is a possibility that if the ore sorting XRF sensors are sufficiently sensitive that high acid consuming gangue minerals, such as calcite, that contain minimal uranium could be rejected from the plant feed. This would allow either reduced acid requirements (and lower operating costs) or increased throughput as the process is acid constrained (ie, there is an onsite acid plant with a fixed production rate and ore feed is at times limited by the acid demand of the ore).
A second area that may benefit from ore sorting is in the criteria used for local exploration. Previously, all exploration was focused on discovering high-grade ores like Kayelekera (~1,000 ppm U3O8). If ore sorting is proven to be successful and the costs are relatively low, then discovering a large medium-grade ore body (~400-800 ppm U3O8) becomes an attractive option as the ore sorting process will convert this into a high-grade feed for the Kayelekera processing plant.
Scalable production potential
In August 2020, ASX-listed Bannerman Resources Limited released details of a Scoping Study for a downsized 8 million-tonne-per-annum (mtpa) development of its flagship Etango Project in Namibia. The study, now coined Etango-8, provides an alternative, and streamlined approach to the superseded, and significantly larger 20 mtpa development assessed to the Definitive Feasibility Study (DFS) level in 2015. Reducing the throughput dramatically reduces upfront capital requirements from US$720 million to $254 million in the 2020 Scoping Study, thereby increasing the investment appeal to financiers.
Under Bannerman Resources’ revised Etango-8 Project, average annual uranium production is reduced from an average 7.5 to 3.5 million pounds U3O8. As well as enabling the company to focus on mining the shallowest and highest-grade ore at Etango, Bannerman has preserved the option for modular expansion toward the original production capacity should demand for those pounds justify rampup.
This could materialise as a rewarding strategy for Bannerman Resources, especially considering the relatively low nature of contracting activity in today’s term market. That is to say that bigger is not always better, despite some prospective producers continuing to promote production schedules of almost 30 million pounds U3O8 per annum. It is important to note that Cameco has yet to market their 18 million-pound-per-annum McArthur River/Key Lake operation. The same applies regarding other idle operations, including Paladin Energy’s Langer Heinrich Mine in Namibia and every pure-play uranium project in the USA. By downsizing Etango’s mill throughput, Bannerman has made its marketable and saleable contract volumes more manageable, potentially improving the agility of Etango to respond more readily to market appetite once it emerges.
By-product production potential
Other metalliferous minerals will almost always be present within the principal uranium-bearing ore. If these minerals have commercial value, and they exhibit the requisite properties for processing (and they exist in economic concentrations), then they too may be mined as by-products of uranium production.
Added to that, during times of low uranium prices, commodity diversification can also present welcome economic support for (re)emerging uranium producers. Bonus elements occurring as by-products to uranium production often result in a proportional increase in revenue at almost no increase to total operating costs. Innovative technologies are transforming the metal and minerals markets, and consequently, certain uranium producers are adapting their marketing strategies and processing pathways to attract investors seeking commodity diversification coupled with notable price potential. Moreover, as concerns over security of supply increase, global demand for base, battery, and critical minerals presents a potential opportunity for those uranium producers competing in a highly competitive pool of prospective producers.
As part of Australian-focused Vimy Resources Ltd’s 2018 DFS, the company investigated a stand-alone base metals plant designed to recover copper, zinc, nickel, and cobalt as mixed sulphide by-products from the tailings of the uranium plant. Following those investigations, the 2018 DFS focused solely on uranium due to low prevailing base metals prices at the time. However, in February 2021, and prompted by growing demand for clean energy solutions and a significant rise in base metals prices, Vimy revisited the viability of the base metals plant. Results now suggest materially improved economics during the first eight years of production when the base-metal bearing deposit, Ambassador, is mined. According to Vimy, at current long-term base metal prices, a LoM base metals by-product credit of around $4.00 to $4.50 per pound of U3O8 produced.
Similarly, US Returning Producer Energy Fuels is the 100 per cent-owner of White Mesa Mill, the only operating conventional uranium processing facility in the USA. White Mesa Mill, located in Utah, can be fed by surrounding projects in the State of Colorado, Utah, Arizona, and New Mexico, as well as by ore purchases or toll milling arrangements with third parties. The White Mesa Mill is predominantly a uranium recovery facility. However, in the wake of low uranium prices, White Mesa Mill has undergone a series of upgrades – starting in 2018 and continuing through 2020 – enabling it to recover other critical minerals like rare earth elements (REEs) from alternate feed sources, and vanadium pentoxide (V2O5) from existing tailings pond solutions.
In February 2019, Energy Fuels began ramping up V2O5 production and selling its product (as ferrovanadium) into the steel industry. Then, in 2020, the company began evaluating alternate feed materials for the recovery of REEs in addition to uranium. In December 2020, a three-year supply contract was agreed with The Chemours Company to acquire natural monazite sands; a by-product of heavy mineral sands mined in the southeast USA and one of the highest-grade REE minerals in the world. And in March 2021, the company embarked on a new REE production initiative with Neo Performance Materials spanning European and North America critical material supply chains.
Typical monazite ore has a higher value than other rare earth bearing ores, as it contains 50-60 per cent total rare earth content, in addition to 0.20-0.30 per cent recoverable natural uranium, which is the typical grade of uranium found in uranium mines that have historically fed the White Mesa Mill. Therefore, in addition to extracting the high concentrations of rare earths from the monazite ore, White Mesa Mill will also extract the naturally occurring uranium in this ore.
TradeTech’s Production Cost Indicator (PCI™)
There is no doubt that the world does need new uranium mines, but by the same token, and despite several companies purporting misleadingly low uranium production costs, the overwhelming majority of uranium miners need higher uranium prices to incentivise operational readiness.
The endgame for a uranium producer is to physically produce profitable pounds of uranium concentrates for its customers and shareholders. At current prices, the only viable conduit by which to achieve that is lowering production costs through innovation and optimisation; to de-risk technical viability, to improve environmental, social, and governance objectives, and to incentivise financing. All of which should culminate in winning long-term contracts with nuclear utilities.
However, in their quest to remain competitive, future uranium producers continue to publish economic studies that emphasise debatably low uranium production costs. Despite contrived attempts by select producers to avoid disclosing ulterior costs, like financing, exploration, legislative, extraordinary, and overhead charges, TradeTech recognises that cash operating/mine-gate (C1), all-in sustaining (AISC), and all-in (AIC) cost metrics do not accurately reflect the true full cost (C3) of doing business in the uranium industry, especially over the complete life-cycle of a mine, from grassroots exploration through to decommissioning, rehabilitation, and restoration.
Although the Long-Term U3O8 Price Indicator and production economics are not (historically) correlated, in April 2020, TradeTech acknowledged a growing disconnect between the LoM full cost (LoM C3) incurred in producing one pound of U3O8 versus its sale price. This prompted the development of a new indicator that accurately captures the true cost of bringing future uranium concentrates to market; in other words, to bridge the gap between a suite of uranium producers’ cost disclosures versus TradeTech's price indicators, which are based on monthly transactional data.
Figure 6 shows TradeTech’s Exchange Value (monthly U3O8 spot price indicator), the Long-Term U3O8 Price Indicator, and Production Cost Indicator (PCI™). The PCI™, in blue, reflects TradeTech’s judgment of the LoM full cost necessary to incentivise and support new primary uranium production needed to sustain the global nuclear fuel industry. Just as TradeTech’s uranium price indicators ebb and flow to reflect transactional data, the PCI™ moves to reflect the rational, weighted average, break-even cost of uranium production needed to sustain reactor requirements ahead.
TradeTech’s PCI™ dispels the notion that the C1, AISC, and AIC cost metrics are comparable. It takes an important step toward providing an indicator that reflects the true cost of producing one pound of uranium derived from extractives technologies being used today and being developed for the future.
The disconnect between production costs, realised uranium prices, and future sales prices is complex; it is one that has become fraught with myth and misunderstanding as producers become frustrated by persistently low prices despite successional cuts to uranium production. Nevertheless, TradeTech believes that complex information from uranium asset evaluations, resources geology, extractives technologies, and mining economics can be distilled down to one readily available, transparent metric.
At its launch in April 2020, the PCI was $44.00 per pound U3O8. The marginal decline in the PCI™ over the last 12 months reflects a combination of circumstances affecting the future supply/demand dynamic. And despite juniors promoting optimistic costs in feasibility studies, TradeTech’s proprietary cost analysis of full costs (C3) necessary to support future production remains well above today’s uranium prices.
This is an irrational state for the uranium market to be in where a paradoxical relationship now exists between supply and demand. However, this relationship cannot coexist for long. Because despite innovation and technology driving costs down, in the past four years, uranium market fundamentals point to a perpetuating supply deficit culminating in a shortfall of over 40 million pounds U3O8 in 2020. Despite that, contracting activity by nuclear utilities continues to reflect a lack of anxiety surrounding the perceived availability of future supply. And this discrepancy has manifested as a gulf between price and cost (Figure 6).
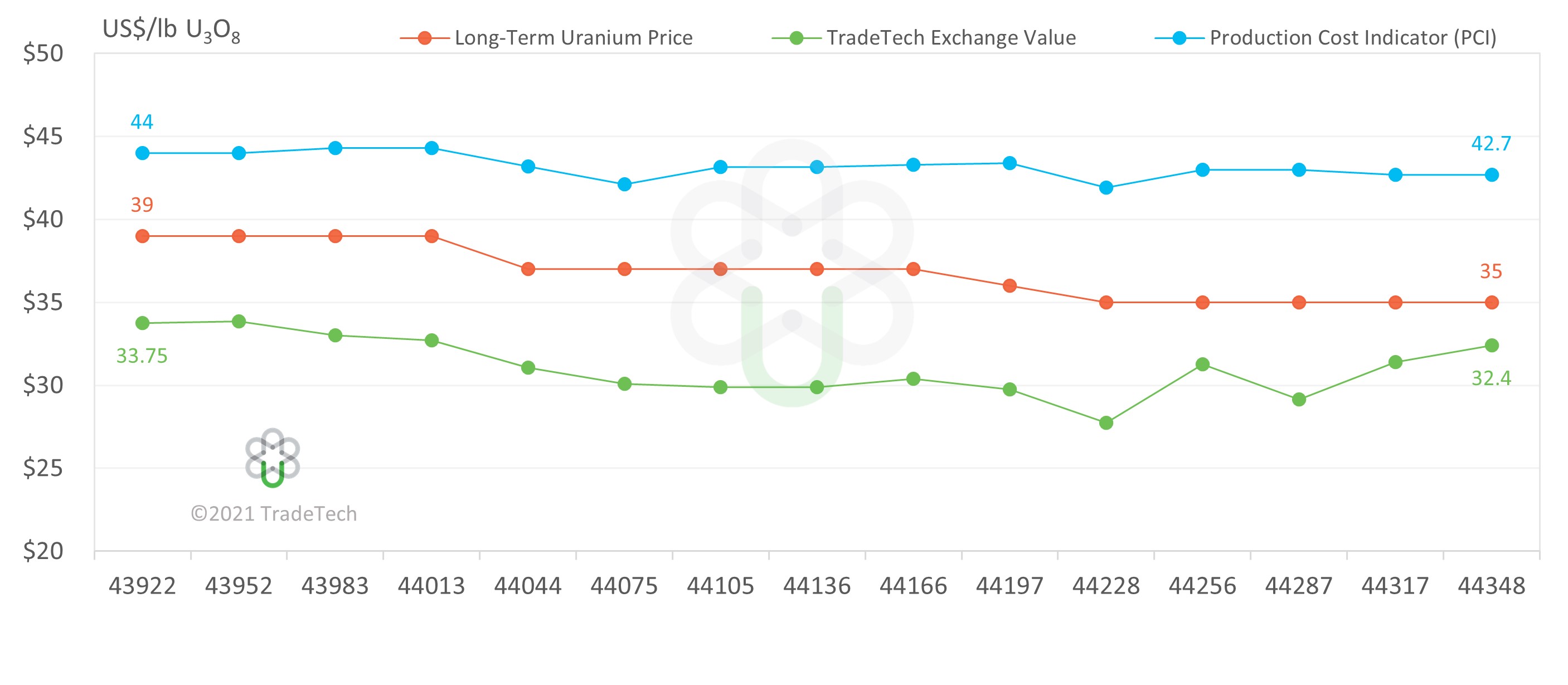
Today’s prevailing price levels might best be characterised as sentiment driven, and the prevailing sentiment for some time in the market has been uncertainty. However, there is reason for optimism – disruptive times tend to foster opportunistic behaviours, which over time are likely to contribute to a realignment of prices with optimised costs that help secure supply well into the future.
Conclusion
Innovation is not simply restricted to technology and changes in the way that uranium is mined and processed; it can apply to a producer’s operating structure, team management, business strategy, and shareholder engagement.
However, the way in which uranium is being mined is changing. The inception of large-scale, low-cost ISR production in Kazakhstan in the early 2000s, and its potential impact on prices ahead, is a significant factor distinguishing the uranium industry from other mineral commodities and investment cycles.
There is no doubt that the world does need new uranium mines. A proportion of new production over the coming decade will undoubtably stem from advanced exploration assets in Kazakhstan. However, the need for end users to maintain a diverse supply portfolio has rarely been more critical than it is today, especially in a geopolitical landscape becoming increasingly sensitive to trade policies and tensions beyond the control of both nuclear utilities and uranium producers.
All considered, technology and innovation represent a viable conduit for the uranium production sector to develop and implement new approaches and processes to realise mineral resource opportunities while improving environmental performance and meeting social expectations.
Still, so long as abundant economic uranium reserves in Kazakhstan exist, future uranium producers competing for sales contracts will find themselves striving to develop better mining technology; technology that reduces operational risk, incentivises capital investment, improves efficiencies at scale, optimises production economics, and aligns a project’s cost competitiveness with the lowest-cost uranium producers.
Just as the market witnessed increasing ISR output in Kazakhstan over the past two decades, this next decade will likely be defined by new technologies. For those newly emerging uranium projects that do not have economies of scale, they must adapt and seek alternative means to lower capital and operational costs over the planned LoM. Future uranium projects that will help sustain the global nuclear fuel industry have already been discovered, delineated, defined, and developed. The geology of those deposits will not change, so technology is now tasked with converting those identified resources, of which there are plenty, into economic uranium reserves.
"Behavioral economics suggests we tend be more irrational when operating in an aroused or emotional state caused by hunger, anger, or frustration (Dan Ariely, 2008). Reason for optimism can be found as disruptive times tend to support greater technological innovation. Hence, we need to stay fed, remain calm, and invest in science and technology to address some of our most pressing problems."